Designing EV Batteries with Lithium-Metal Anodes
Lithium metal anodes promise theoretical capacities of 3860 mAh/g—ten times that of conventional graphite—but face significant challenges in practical implementation. Current designs struggle with dendrite formation during cycling, volumetric expansion exceeding 100%, and rapid capacity fade due to continuous solid electrolyte interphase (SEI) formation.
The fundamental challenge lies in stabilizing the highly reactive lithium metal surface while maintaining the high ionic conductivity needed for practical charge/discharge rates.
This page brings together solutions from recent research—including three-dimensional architectures with conformal protection layers, composite protective films combining ionic and electronic conductivity, and novel surface modification approaches using carbon-based materials. These and other approaches focus on achieving stable cycling while maintaining the high energy density advantage of lithium metal.
1. Negative Electrode with Micron-Scale Particle Protective Layer for Lithium Metal Batteries
SAMSUNG ELECTRONICS CO., LTD, 2024
Negative electrode for lithium metal batteries with improved cycle life and reduced volumetric change during charging. The negative electrode has a protective layer on the lithium metal surface with particles sizes between 1-100 microns. The protective layer has a Young's modulus of 106 Pa or greater. This provides mechanical strength to prevent dendrite growth and volumetric expansion during charging. The protective layer also improves lithium deposition density compared to bare lithium metal electrodes.
2. Lithium Metal Composite Electrode with In Situ Grown Conductive Layer and 3D Framework Structure
Contemporary Amperex Technology Co., Limited, 2024
Lithium metal composite electrode material for lithium metal batteries with improved cycle stability and reduced dendrite formation compared to conventional lithium metal electrodes. The composite electrode material has a lithium-containing conductive layer grown in situ on the surfaces of lithium metal particles. This layer isolates the lithium metal from the electrolyte to reduce irreversible reactions and dendrite growth. The layer includes an inorganic lithium compound and lithium alloy. The layer serves as a 3D framework structure that coats the lithium metal particles. This framework reduces volume expansion and dendrite formation during cycling. The composite electrode material is prepared by mixing lithium metal, a metal compound, and conductive carbon, then heat treating to grow the in situ layer.
3. Lithium Metal Electrode with Porous Carbon Layer Formed by Gas Desorption for Dendrite and Side Reaction Mitigation
CONTEMPORARY AMPEREX TECHNOLOGY CO., LIMITED, 2023
A lithium metal negative electrode for lithium-ion batteries that addresses the issues of dendrite formation and interface side reactions. The electrode has a porous carbon layer with pores formed by desorbing adsorbed gas during slurry coating. This creates a 3D pore structure in the carbon layer that prevents dendrite growth and reduces side reactions compared to a smooth carbon layer. The pore structure allows lithium metal to deposit inside the pores instead of on the surface, reducing dendrite formation. The pores also provide a pathway for lithium ion transfer, mitigating side reactions.
4. Batteries with Lithiated Carbon-Coated Lithium Anodes, Sulfurized Carbon Cathodes, and Graphene Nanoribbon-Modified Separators
WILLIAM MARSH RICE UNIVERSITY, 2023
Batteries with improved safety, cycling stability, and energy density by using lithiated carbon-coated lithium metal anodes, sulfurized carbon cathodes, and graphene nanoribbon (GNR) modified separators. The lithiated carbon coating on lithium metal prevents dendrite formation during charging, the sulfurized carbon cathodes have high capacity and reduced polysulfide shuttle, and the GNR-modified separators prevent sulfur migration.
5. Lithium Metal Battery with Positive Electrode Cross-Section Equal to or Larger Than Negative Electrode
LG ENERGY SOLUTION LTD, 2025
Lithium metal battery with improved energy density and safety compared to traditional lithium-ion batteries. The lithium metal battery has a unique design where the cross-sectional area of the positive electrode is equal to or larger than that of the lithium metal negative electrode. This prevents issues like lithium dendrite growth and short circuits that can occur when the negative electrode has a larger area. The equalized electrode areas improve battery performance and safety.
6. Stable Solid Electrolyte Interphase in Cylindrical Anode-Free Li-Metal NMC90 Batteries with Li<sub>2</sub>NiO<sub>2</sub> Prelithiation and Fluorine-Rich Electrolytes for High Energy Density
thitiphum sangsanit, ronnachai songthan, surat prempluem - American Chemical Society, 2025
This study advances anode-free lithium-metal batteries (AFLMBs) by integrating nickel-rich NMC90 cathodes and fluorine-rich electrolytes in large-format 18650 cylindrical cells. A key innovation is the incorporation of 10 wt % Li-rich Li2NiO2 as a prelithiation agent cathode, which mitigates initial lithium-loss improves Coulombic efficiency. The electrolyte includes 30% (v/v) fluoroethylene carbonate (FEC) cosolvent, suppresses inactive lithium deposition stabilizes solid interphase (SEI). Unlike conventional AFLMBs that require external pressure, this work uses stainless-steel casing with tailored jelly roll configuration to mechanically regulate plating. optimized cells deliver an energy density 320 Wh/kg, maintain stable cycling over 140 cycles, support 4C-rate operation. Post-mortem analysis reveals LiF-rich SEI extends cycle life, while operando X-ray diffraction provides insights into structural evolution. research offers scalable strategy for high-energy through synergy prelithiation, design, mechanical stabilization.
7. Composite Metal Foil with Lithiophilic Layer for Lithium Metal Batteries
INDUSTRIAL TECHNOLOGY RESEARCH INSTITUTE, 2025
Composite metal foil for lithium metal batteries with improved lithium plating properties to enhance battery performance. The composite foil has a first metal layer (copper, nickel, stainless steel) and a second metal layer with a contact angle to lithium metal lower than 90 degrees. This lithiophilic second layer promotes favorable lithium nucleation and deposition morphology compared to bare first layer foils.
8. Composite Separator with MXene-Coated Polymer Film for Lithium Metal Batteries
DREXEL UNIVERSITY, 2025
A composite separator material for lithium metal batteries that improves stability and prevents dendrite growth during cycling. The composite separator has a polymer film coated on at least one side with a MXene material, a two-dimensional transition metal carbide, nitride, or carbonitride. The MXene coating promotes uniform lithium nucleation, stable SEI formation, and suppresses dendrite growth compared to uncoated separators.
9. Porous Ceramic Electrolyte with Intermetallic Coating for Solid State Batteries
GE INFRASTRUCTURE TECHNOLOGY LLC, 2025
Solid state batteries with reduced dendrite formation and voids in lithium metal anodes, improved energy density, and simplified manufacturing compared to conventional solid state batteries. The key innovation is a porous ceramic electrolyte with a low porosity (10-80%) and an intermetallic coating on the surface. The interconnected porous structure allows lower current densities and reduced dendrite growth, while the intermetallic layer stabilizes the lithium-electrolyte interface and directs lithium growth. This mitigates void formation and prevents short circuits. The lower porosity enables thinner electrolytes for higher energy density. The intermetallic coating also facilitates lithium transport and interfacial contact. The porous electrolyte can be formed by sintering, coating, or graded deposition methods.
10. Coordination‐Induced Plastic Ceramic‐Ether Coupling Electrolyte for High‐Voltage Lithium Metal Batteries
yanan yang, zhiqian hou, dezhi yang - Wiley, 2025
Abstract The highvoltage reactivity and flammability of electrolytes remain critical challenges for highsafety highenergydensity lithium metal batteries (LMBs). Here, a novel ceramicether coupling electrolyte (CCE) is reported, in which thin liquid layer the ether immobilized on particle surface Li 6.4 La 3 Zr 1.4 Ta 0.6 O 12 (LLZTO) matrix through coordination interactions. With an LLZTO content exceeding 82%, it demonstrates high plasticity, nonflammability, oxidation voltage threshold above 5.0 V. strong interactions between solvent molecules or anions are revealed, generate cohesive forces that impart highplastic rheological behavior to matrix, ensuring conformal contact at solid/solid interfaces. These also lead loose + solvation sheath electrolyte, not only accelerates transport, achieving ionic conductivity 2.7 10 4 S cm 1 , but promotes anion decomposition form inorganicrich cathode interphase (CEI). This enables Li/LiNi 0.8 Co 0.1 Mn 2 (NCM811) cells operate stably cutoff 4.5 work can open up new insights into design safety LMBs.
11. Electrolyte Composition with Iodine and Fluorosilicate Additives for Enhanced Solid Electrolyte Interphase Stability in Metal-Ion Batteries
SILA NANOTECHNOLOGIES INC, 2025
Electrolytes for metal-ion batteries like lithium-ion batteries that improve stability and performance of high capacity electrodes like silicon anodes. The electrolytes contain additives like iodine, iodine-containing salts, and fluorosilicates to enhance solid electrolyte interphase (SEI) stability and Coulombic efficiency (CE). These additives reduce solvent permeation, improve mechanical properties, and facilitate lithium transport through the SEI. They also reduce dendrite growth on lithium metal anodes. The electrolytes can contain multiple salts like lithium salts, alkaline earth salts, and fluorosilicates. The total concentration of additives is optimized for performance.
12. Freestanding Amorphous Sulfide Glass Solid Electrolyte Sheets with High Lithium Ion Conductivity
POLYPLUS BATTERY CO, 2025
Standalone solid electrolyte sheets for lithium batteries that are freestanding, amorphous, and highly lithium ion conductive to enable high performance lithium batteries without the need for a liquid electrolyte. The solid electrolyte sheets are made from continuous sulfide glasses with intrinsic room temperature lithium ion conductivity above 10^-4 S/cm. The glass sheets have parallel edges, battery size, and are self-supporting. The sulfide glass composition and processing are optimized for interfacial stability with lithium metal. The continuous glass sheets can be cut into separators for battery cells or used as substrates for electrode assemblies.
13. Lithium Metal Anodes with Two-Dimensional Transition Metal Nitride and Carbide Nanocrystal Framework
DREXEL UNIVERSITY, 2025
Stable lithium metal anodes for Li-ion batteries that overcome the issues of dendrite growth during cycling. The anodes use free-standing films of two-dimensional arrays of transition metal nitride and carbide nanocrystals as the framework for Li deposition. The interconnected few-nanometer nanocrystals provide high surface area, fast electron/ion transport, and strong interaction with polysulfides for stable sulfur cathodes. The films can be made by reacting transition metal precursors on salt templates with ammonia.
14. Battery Cell with Lithiophilic Metal Oxide Coated Current Collector for Lithium Metal Anodes
GM GLOBAL TECHNOLOGY OPERATIONS LLC, 2025
Battery cell with improved strength and handling properties for lithium metal anodes using a lithiophilic metal oxide coating on the current collector. The coating helps bond the lithium metal to the collector during manufacturing and operation, preventing voids and delamination. It also increases the strength of the anode without adding weight or resistance like alloying or thicker mesh. The coating material can be selected from zinc oxide, indium oxide, tin oxide, bismuth oxide, or aluminum oxide. The coated collector is then sandwiched between lithium metal layers to create the anode electrode.
15. Calendering Process for Flattening Lithium Metal Coatings on Battery Anode Foils
LI-METAL CORP, 2025
A process to improve the surface of lithium metal coatings on battery anodes for better performance. The process involves calendering the lithium-coated anode foil to flatten the lithium surface and make it parallel with the foil surface. This is done after depositing the lithium and before exposing it to air. Calendering converts the convex lithium surface to a flat, planar surface on the foil. This provides a superior surface for the lithium anode in batteries compared to the original convex lithium layer.
16. Lithium Metal Battery with Boron Nitride-Infused Protective Film and Gel Polymer Electrolyte
SAMSUNG SDI CO LTD, 2025
A lithium metal battery with improved cycle life and reduced dendrite growth by using a protective film containing boron nitride (BN) and a binder on the anode current collector. The gel polymer electrolyte contains BN, a nitrile-based compound, and a liquid electrolyte. The absence of BN in the protective film and lack of BN and nitrile compounds in the electrolyte led to degraded performance due to dendrite growth. The BN in the protective film suppressed dendrite formation and the BN and nitrile compounds in the electrolyte improved ionic conductivity.
17. Single-Layer Anode with Integrated Current Collector Comprising Carbon-Silicon-Metal Oxide Composite
NORTHVOLT AB, 2025
Single-layer anode for a lithium-ion battery that eliminates the need for a separate current collector, reducing weight and volume. The anode active material itself functions as the current collector. The active material contains carbon, silicon, and/or metal oxide without an additional metal foil or grid. This allows lithium plating to occur inside the porous anode material rather than on a separate current collector. The anode has a porosity of 10-90% to facilitate lithiation and plating. The single-layer anode improves cycling stability and reduces the risk of cell failure compared to conventional multi-layer anodes.
18. Solid-State Battery with Coated Current Collector Featuring Hard Metal Layer on Soft Metal Substrate
SAMSUNG SDI CO LTD, 2025
A solid-state battery with improved cycle life by preventing short circuits. The battery has a negative electrode with a coated current collector. The collector has a softer metal substrate covered by a harder metal layer. This reduces scratches on the substrate during cycling. The harder layer prevents irregularities in the lithium plating that cause cracks in the solid electrolyte. This reduces the risk of short circuits. The battery also uses a sulfide-based solid electrolyte to further prevent substrate degradation.
19. Battery with Aluminum Anode Current Collector and Layered Structure for Enhanced Conductivity and Adhesion
AISAN KOGYO KABUSHIKI KAISHA, TOYOTA JIDOSHA KABUSHIKI KAISHA, 2025
Battery design with an aluminum anode current collector to improve energy density and heat dissipation, without degrading discharge properties. The aluminum anode reacts faster than some other anode materials due to lower reaction potential, leading to alloying and damage. To prevent this, the aluminum anode is covered with layers. An intermediate layer between the aluminum and anode active material layer provides adhesion and conductivity. Alternatively, the anode active material layer itself is a concentration gradient with more resin towards the aluminum side. This supports adhesion and conductivity, decreases electric resistance, and inhibits aluminum reaction with lithium.
20. Solid-State Electrolyte with Multiple-Doped LixTiyMm(PO4)3 Composition for Lithium Metal Anodes
NATIONAL TSING HUA UNIVERSITY, 2025
Solid-state electrolyte for lithium-ion batteries that reduces deterioration of the electrolyte during cycling when used with lithium metal anodes. The electrolyte contains a multiple-doping material with a composition LixTiyMm(PO4)3 where 0.85≤x≤1.5, 0<y≤0.6, M is at least three different doping elements, and 1.2≤m≤1.7. The doping elements partially substitute for titanium to reduce its concentration. This prevents reaction of titanium ions with lithium metal anodes that causes deterioration. The substitution keeps ionic conductivity for efficient battery operation. The doping elements have similar oxidation states and ionic radii to titanium for stability.
Get Full Report
Access our comprehensive collection of 109 documents related to this technology
Identify Key Areas of Innovation in 2025
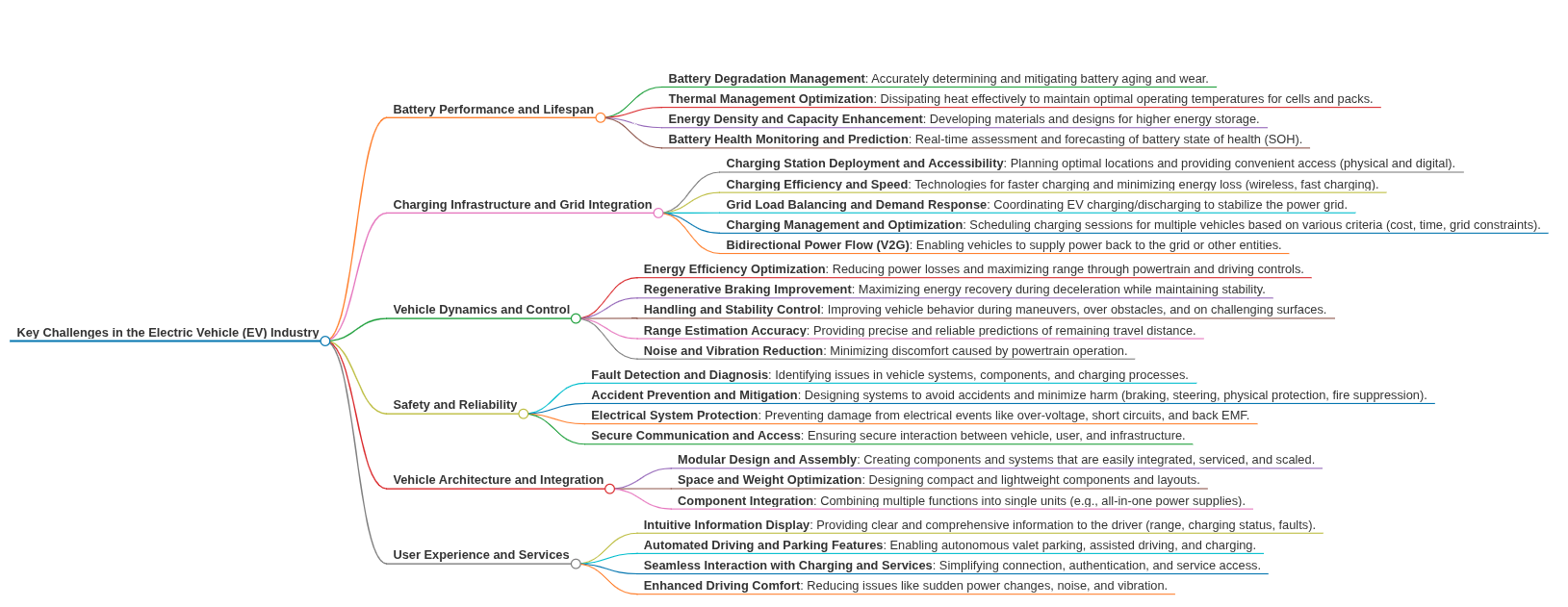