Interface Stability in Solid-State Batteries
Solid-state batteries promise higher energy densities and improved safety, but interface degradation remains a critical barrier. At the electrode-electrolyte boundaries, mechanical stress from volume changes during cycling can create voids and cracks, while chemical instabilities lead to increasing interfacial resistance. Current interfaces typically show degradation after 100-1000 cycles, with contact losses exceeding 20% of the original interface area.
The fundamental challenge lies in maintaining stable mechanical and chemical contact between solid electrodes and electrolytes while accommodating the volume changes and electrochemical reactions that occur during battery operation.
This page brings together solutions from recent research—including protective interface layers with controlled Young's modulus, electrode designs that minimize volumetric expansion, novel coating architectures for resistance management, and structural approaches to maintaining contact under cycling stress. These and other approaches focus on achieving the interface stability needed for commercially viable solid-state batteries.
1. Porous Battery Active Material with Li22Si5 Crystal Phase for Volume Stabilization
TOYOTA JIDOSHA KABUSHIKI KAISHA, 2024
Reducing volume change of battery active materials during charging/discharging by creating a porous structure inside the material particles. The method involves preparing a LiSi precursor with a specific crystal phase, then extracting Li using a solvent to form voids in the precursor. This step creates a porous active material with reduced volume change compared to non-porous materials. The specific crystal phase is Li22Si5.
2. All Solid State Battery with Selective Anode Coating for Resistance Distribution Control
TOYOTA JIDOSHA KABUSHIKI KAISHA, 2024
An all solid state battery design to prevent short circuits in the anode during charging by controlling the resistance distribution. The battery has a coating layer with lithium titanate on the anode current collector. The coating exists in the region where the anode and cathode are opposing but is omitted in the region where they are not opposed. This helps balance charge reaction progression in both regions. In the opposed region, the coating provides a conductive path to lower anode potential. In the non-opposed region, the coating omission reduces resistance compared to the coated region. This prevents uneven charge reaction progression and minimizes short circuits in the anode.
3. Lithium Nickel-Based Oxide Positive Electrode Material with Zirconium-Enriched Surface Layer
UMICORE, 2024
Lithium nickel-based oxide positive electrode material for solid-state batteries with improved first charge capacity. The material contains Li, Ni, Mn, Co, D, and Zr oxides. The Ni content is 50-85%, Mn and Co are 0-40%, D is 0-2 mol % of other elements, and Zr is 0.1-5 mol %. The Zr content in the surface layer is around 0.1-0.5 at %. This composition and Zr surface enrichment provide a high first charge capacity of at least 160 mAh/g in solid-state batteries.
4. Lithium Nickel-Based Oxide Electrode Material with Zirconium Doping and Specific Nickel Content for Solid-State Batteries
UMICORE, 2024
Lithium nickel-based oxide positive electrode active material for solid-state batteries in electric vehicles with improved charge capacity. The material contains nickel, cobalt, manganese, optional dopants like aluminum or boron, and zirconium. The nickel content is 50-75 mol %, zirconium is 0.1-5 mol %, and the zirconium content in the surface layer is around 0.1 at %. This composition improves the first charge capacity to at least 160 mAh/g in solid-state batteries. The zirconium-doped lithium nickel oxide provides a higher charge capacity compared to traditional lithium nickel oxide materials in solid-state batteries.
5. Composite Interlayer with Lithium Nitrate and Organic Matrix for Lithium Metal Solid-State Batteries
GM GLOBAL TECHNOLOGY OPERATIONS LLC, 2024
A composite interlayer for lithium metal solid-state batteries to improve cycle life and reduce impedance at the lithium metal/solid electrolyte interface. The interlayer is formed by coating the lithium metal with a mixture of lithium nitrate, dimethoxyethane, and trimethyl phosphate. This coating is applied to the lithium metal for 1-2 hours, then dried to form the interlayer between the lithium metal and solid electrolyte. The interlayer contains an ionic conductor, like lithium nitrate, dispersed in an organic matrix. This composite interlayer suppresses side reactions between lithium metal and the solid electrolyte, reducing impedance, and improves cycle life compared to bare lithium metal.
6. Composite Electrolyte with Inorganic Solid Electrolyte in Polymer Matrix for Lithium-Ion Batteries
QuantumScape Battery, Inc., 2024
Composite electrolytes for lithium-ion batteries with improved stability against dendrite growth and resistance to cracking when used with high-capacity lithium metal anodes. The composite electrolytes have a high volume fraction of inorganic solid electrolyte embedded in an organic polymer matrix. The inorganic component provides ionic conductivity while the polymer prevents dendrite growth and cracks. The composite electrolytes have fracture strengths between 5-250 MPa. The inorganic material can be a lithium-stuffed garnet oxide or antiperovskite oxide. The organic polymer can be entangled with a surface species on the inorganic particles. The composite electrolytes prevent dendrite formation and cycling at high current densities without cracking compared to pure organic electrolytes.
7. Battery Cell with Main Body and Compressible Buffer Pad Featuring Matched Thickness Regions
CONTEMPORARY AMPEREX TECHNOLOGY CO., LIMITED, 2024
Battery cell design to improve interface wrinkling, expansion force, and safety during charging and discharging. The cell has a main body with thinner and thicker regions. A compressible buffer pad is wrapped around the main body. The pad has thicker and thinner sections matching the main body thicknesses. This provides a uniformly thick cell with the thinned regions filled. It prevents wrinkling during expansion. The pad compresses to absorb expansion force and reduce stress between cells.
8. Three-Layer Lithium Battery Separator with Ceramic-Coated Polymer Core
University of Dayton, 2024
Lithium battery separator that combines the benefits of polymer separators and solid ceramic electrolytes for improved battery performance. The separator is a three-layer structure with ceramic electrolyte coatings on either side of a polymer separator. The ceramic layers, made of materials like lithium aluminum germanium phosphate (LAGP), provide high ionic conductivity, stability, and prevent dendrite formation. The polymer separator provides flexibility and mechanical strength. The hybrid separator shows better electrolyte uptake, ionic conductivity, interface stability, cycle life, and voltage polarization compared to regular polymer separators.
9. Continuous Fabrication Process for All-Solid-State Batteries with Zig-Zag Stacked Bendable Electrodes
GM GLOBAL TECHNOLOGY OPERATIONS LLC., 2024
Scalable continuous fabrication process for all-solid-state batteries using zig-zag stacking of bendable electrodes. The process involves stacking alternating continuous anode and cathode electrodes in a zig-zag pattern instead of punched sheets. This allows scalable production of ASSB cells with improved mechanical flexibility. The continuous electrodes have current collectors and active materials on both sides. A sulfide electrolyte layer is applied between adjacent electrodes. The zig-zag stacking provides high speed, accurate positioning, and reduced cost compared to punching individual sheets.
10. Battery Module with Adjustable Compression Plates for Consistent Cell Tension in Solid State Batteries
Bayerische Motoren Werke Aktiengesellschaft, 2024
A battery module design and bracing method for solid state batteries to maintain consistent cell tension during charging and discharging. The module has variable spacing between compression plates based on cell size changes during charge/discharge. This allows precise adjustment of cell tension in different states to prevent performance degradation. The variable spacing is achieved by having flexible cells that expand/contract during charge/discharge. Compression plates with adjustable gaps compress the cells at all states. This prevents cell buckling or misalignment as the cells expand during charge.
11. Multiphase Thin Film Solid-State Electrolytes with Cubic Lithium-Stuffed Garnet and Secondary Phase Inclusion
QuantumScape Battery, Inc., 2024
Multiphase thin film solid-state electrolytes for solid-state batteries that have improved properties like stability, compatibility with Li metal, density, and strength compared to conventional single-phase garnet electrolytes. The multiphase electrolytes contain a primary cubic lithium-stuffed garnet phase with a secondary phase inclusion. The cubic garnet phase is present at 70-99.9% volume, while the secondary phase is 30-0.1% volume. The multiphase structure provides better properties for solid-state batteries compared to single-phase garnet electrolytes.
12. Composite Solid-State Battery Electrolyte with Sulfide and Polymer Phases Separated by Structured Additive Coating
CONTEMPORARY AMPEREX TECHNOLOGY CO., LIMITED, 2024
Composite solid-state battery electrolyte that improves cycling stability and reduces short circuiting compared to pure sulfide electrolytes. The composite electrolyte has a sulfide electrolyte, a polymer electrolyte, and a functional additive material with a specific structure. The functional additive coats the sulfide electrolyte surface to prevent polymer contact and degradation during battery cycling. The composite electrolyte is made by mixing the sulfide, additive, and solvent, removing the solvent, and then mixing with the polymer electrolyte.
13. Battery Electrodes with Core-Embedded Carbon and Hierarchical Protective Shells on Sulfide Composites
Sila Nanotechnologies, Inc., GEORGIA TECH RESEARCH CORPORATION, 2024
Battery electrodes with improved stability and performance for metal-ion batteries like lithium-ion batteries. The electrodes contain composites of sulfur, lithium sulfide (Li2S), or other metal sulfides, along with conductive carbon. The carbon is embedded in the core of the composite. The electrodes also have a protective layer formed during battery operation via electrolyte decomposition. This layer slows further electrolyte decomposition or reduces reactions between the active material and solvent. The composite formation involves dissolving the sulfide in a solvent, then evaporating it to form particles. The solvent extraction allows uniform size and distribution of the sulfide particles. The hierarchical protective shells on the particles further improve stability by providing multiple levels of protection if outer shells fail.
14. Positive Electrode with Nanometer-Scale Polymer Fibers and Inorganic Particles for Solid-State Batteries
KABUSHIKI KAISHA TOSHIBA, 2024
A positive electrode, electrode, and secondary battery design to improve cycle life, discharge rate, and low temperature performance of solid-state batteries. The electrode contains positive electrode active material particles, polymer fibers with 1-100 nm diameter, and inorganic solid particles. The polymer fibers help prevent expansion/contraction of the active material during charge/discharge cycles, reducing resistance and cycle degradation. The inorganic solid particles further enhance cycle life and performance by reducing electrode-electrolyte interface resistance. The composite electrolyte layer between the electrodes contains nanofiber dispersed in an aqueous electrolyte solution.
15. Positive Electrode Sheet with Core-Shell Structured Active Material and Multi-Layer Configuration
CONTEMPORARY AMPEREX TECHNOLOGY CO., LIMITED, 2024
Positive electrode sheet for high energy density, high rate, long cycle life, and safe secondary batteries. The sheet has a core-shell structured positive electrode active material with an inner core of Li1+xMn1-yAyP1-zRzO4 coated with pyrophosphate and phosphate layers. This improves stability, suppresses manganese dissolution, and promotes lithium ion transport compared to pure LiMnPO4. The sheet can have single or multi-layer construction with the core-shell active material and additional layers of the second positive electrode material like LiNixCobMnO2. The multi-layer configuration allows optimizing properties like rate performance, cycling stability, and safety.
16. Secondary Battery with Thin Negative Electrode Collector and Low Impedance Interface Film
CONTEMPORARY AMPEREX TECHNOLOGY CO., LIMITED, 2024
Secondary battery with high energy density, power performance, and safety. The battery has a thin negative electrode collector to increase capacity, but reduces internal resistance and heat generation compared to thicker collectors. This is achieved by adding a compound between the negative electrode layers that forms a low impedance interface film. The compound composition and negative electrode thickness are optimized to balance capacity increase, interface impedance reduction, and safety. The positive electrode thickness, elongation, and compaction density are also controlled.
17. Lithium Secondary Battery with Electrode Tap Receptor and Gas Barrier Layer
SK ON CO., LTD., 2024
A lithium secondary battery design with improved energy density and durability compared to conventional pouch cells. The battery has an electrode assembly with protruding electrode taps, surrounded by a case and an electrode tap receptor. The receptor houses the protruding taps and has a gas barrier layer to prevent gas ingress/egress. This eliminates the need for sealing protrusions on the case. The barrier receptor improves energy density by avoiding internal space loss. It also prevents gas ingress/egress through the taps, improving stability.
18. Solid Electrolyte Composition with High SP Value Polymer Binder for Enhanced Particle-Collector Interface in All-Solid State Batteries
FUJIFILM Corporation, 2024
Solid electrolyte composition for all-solid state secondary batteries that improves interfacial resistance between solid particles and collectors. The composition contains an inorganic solid electrolyte, binder particles with specific surface properties, and a dispersion medium. The binder particles have a polymer with an SP value of 10.5 cal/cm^3/2 or more and an average diameter of 10-50,000 nm. This composition provides favorable wettability, bonding, and resistance suppression for all-solid state batteries.
19. All-Solid-State Battery System with Isostatic Pressurization via Closed Chamber and Fluid Control Mechanism
Hyundai Motor Company, Kia Corporation, 2023
An all-solid-state battery system that maintains uniform pressure inside the battery during charging and discharging to prevent cell expansion and contraction issues. The system uses a closed chamber filled with pressurizing fluid to apply isostatic pressure to all cells. A state detector monitors battery conditions. A controller adjusts temperature and fluid flow rates to keep pressure within a range based on cell state. This prevents volume changes from affecting cell pressurization.
20. Lithium Battery with Large Particle Size Positive Electrode Material for Enhanced Stability
CONTEMPORARY AMPEREX TECHNOLOGY CO., LIMITED, 2023
Lithium battery with improved cycle life and storage performance at high operating voltages by using a positive electrode material with large particle size to stabilize the interface and prevent deterioration. The positive electrode active material is single crystal or single crystal-like particles with a size of 1-20 μm, preferably 3-15 μm. This large particle size reduces the specific surface area to minimize side reactions at the interface, improving cycle life and storage performance.
Get Full Report
Access our comprehensive collection of 180 documents related to this technology
Identify Key Areas of Innovation in 2025
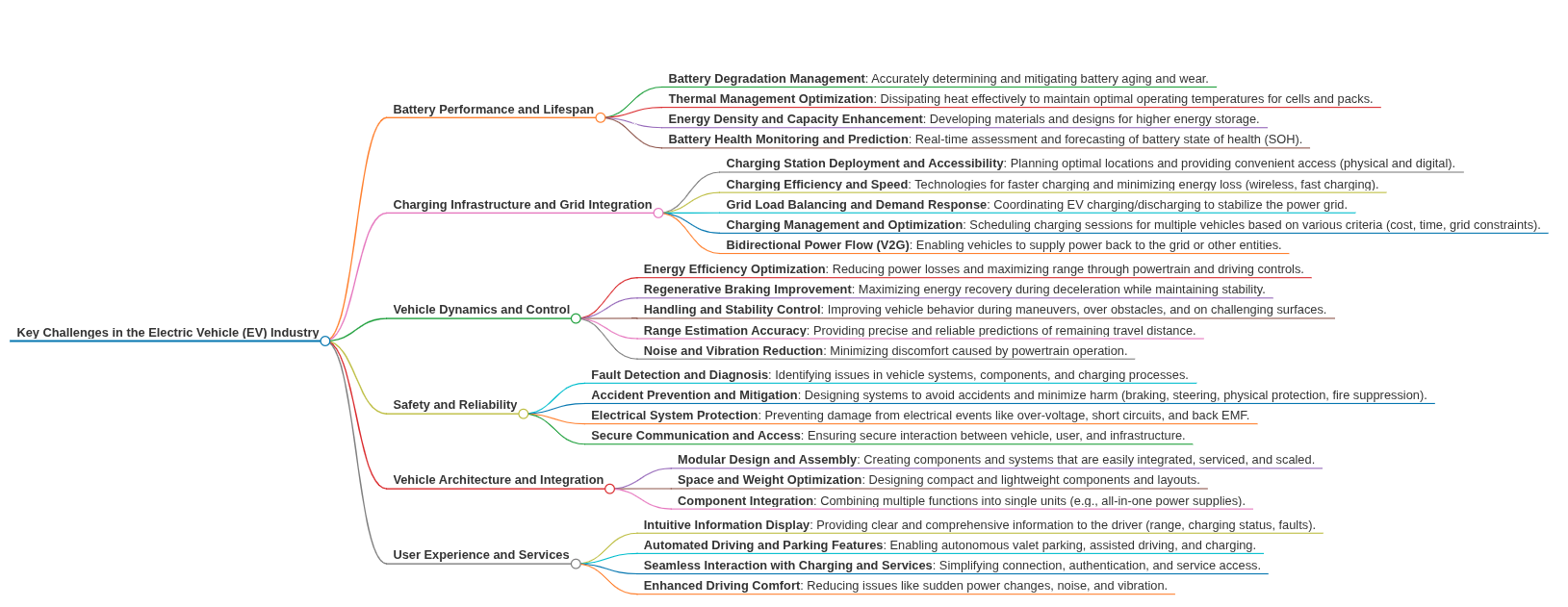