Lithium Metal Vanadium Oxide Batteries for Electric Vehicles
Lithium metal vanadium oxide batteries offer theoretical energy densities above 400 Wh/kg, yet current implementations achieve only 40-60% of this potential due to electrode degradation, dendrite formation, and interfacial resistance losses. At typical charging rates of 0.5-1C, these systems show capacity retention falling below 80% after 500 cycles, with accelerated fade at elevated temperatures above 45°C.
The fundamental challenge lies in balancing the high energy density potential of lithium metal anodes against their tendency toward dendrite formation and reactive degradation during cycling.
This page brings together solutions from recent research—including multi-layer anode architectures with protection layers, elastic polymer interfaces for dendrite suppression, advanced electrolyte formulations, and novel separator designs. These and other approaches focus on extending cycle life while maintaining the high energy density advantages of lithium metal systems.
1. Alkali Metal Battery with Thick Electrodes Formed by Direct Wet Mixture Deposition
HONEYCOMB BATTERY CO, 2024
High energy density alkali metal batteries with thick electrodes and high active material loading. The process involves continuously depositing wet electrode mixtures directly onto the current collectors to form thick electrodes with high active material content. The wet electrodes are then combined to make the battery. This allows thicker electrodes without drying issues or high resistance. The high active material loading and thickness increases volumetric energy density.
2. Alkali Metal Batteries with Thick Quasi-Solid Electrodes and 3D Conductive Network
HONEYCOMB BATTERY CO, 2020
High energy density alkali metal batteries with shape conformability for compact devices and electric vehicles. The batteries have thick electrodes with high active material loading, low overhead weight and volume, and high capacity. The electrodes are quasi-solid with 30-95% active material, 5-40% electrolyte, and 0.01-30% conductive additive. The additive forms a 3D network for electrical conductivity. The thick electrodes are deformable and conformable for compact battery shapes. The batteries can be primary or secondary lithium or sodium cells, lithium-ion, sodium-ion, or lithium-ion capacitors.
3. Non-Aqueous Electrolyte Secondary Battery with Composite Positive Electrode of Lithium-Containing Vanadium Oxide and Lithium Nickel Cobalt Manganese Oxide
SANYO ELECTRIC CO LTD, 2007
Non-aqueous electrolyte secondary battery with improved capacity using a composite positive electrode containing both lithium-containing vanadium oxide (LiV2O5) and another lithium-containing material like LiNi0.4Co0.3Mn0.3O2 or LiFePO4. The composite electrode allows higher battery capacity compared to using just LiV2O5. The LiV2O5 provides high capacity, but forms an amorphous layer that reduces conductivity. The other lithium material helps maintain battery voltage and prevents the formation of the amorphous layer. By using both lithium materials, the battery capacity is increased while avoiding voltage drop issues from using pure LiV2O5.
Get Full Report
Access our comprehensive collection of 3 documents related to this technology
Identify Key Areas of Innovation in 2025
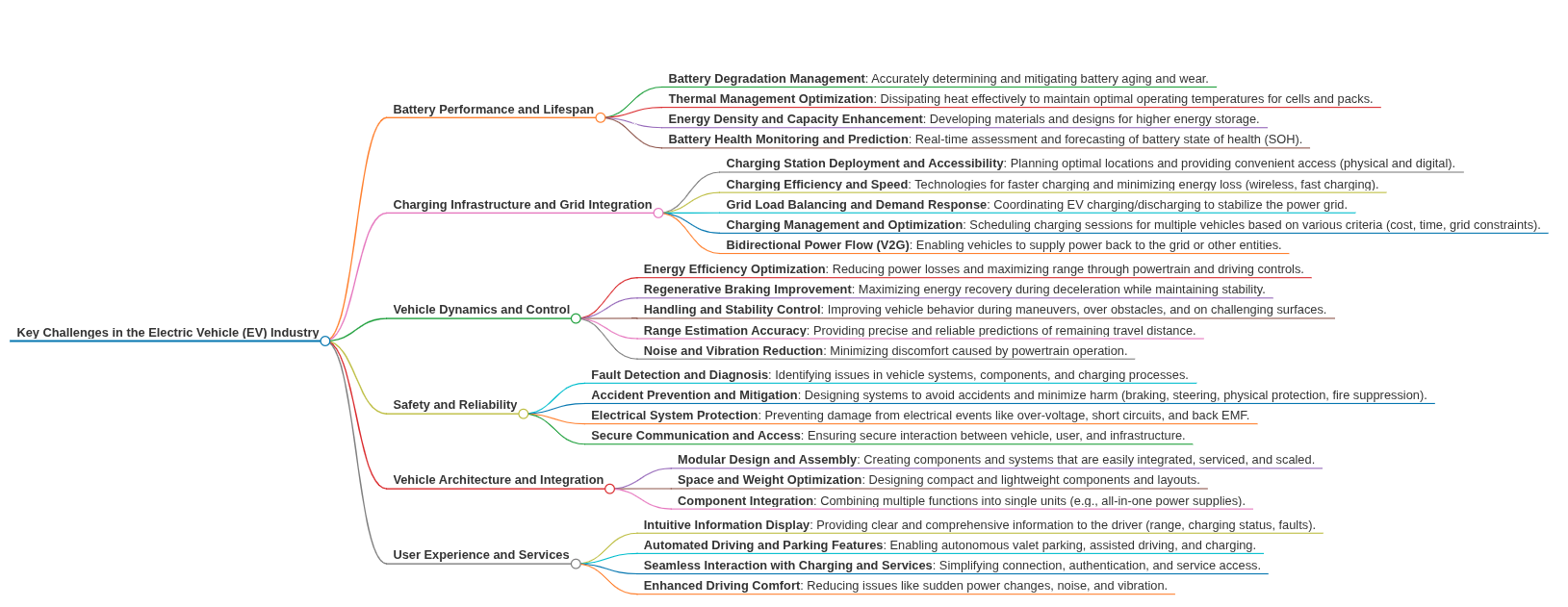